Skin pH plays a critical role in the health of our skin.
Although generally acidic due to the nature of the outermost layer of our skin — the stratum corneum — skin pH levels vary across various parts of our body and in response to the use of certain beauty and personal care products, as well as physiological and other environmental factors, with abnormal pH levels now linked to several skin conditions.
Here we explore some of the key mechanisms responsible for determining skin pH and the link to skin function and health (including the role of the skin microbiome), as well as expanding on the potential effects of cosmetics and cleansing products.
Taking contemporary hygiene practices, such as cleansing with soap and detergents, as an example, we outline how such practices can negatively impact skin pH and play a part in increased rates of skin conditions such as atopic dermatitis. This highlights the need for caution when it comes to choosing which cleansers and other personal care products to use as part of our daily routines.
Skin pH and health — what do we know so far?
The normal range for skin surface pH is 4.1–5.8, with slight variations at different points across the body [1,2] (see our pH scale in Fig. 1, showing the pH of skin and various personal care products). Facial skin is generally acidic with a low pH, while skinfold sites, such as the armpit and groin, have relatively high pH levels — a characteristic that may affect the microbiome and could account for increased occurrence of skin infection and allergic reactions (for example, leading to development of eczema) in these areas [3]. A stable and more acidic skin pH, by contrast, supports the local skin microbiome, which can subsequently provide immune-system-boosting properties to the skin and help to regulate the skin structure and function [4].
Science has demonstrated a link between abnormal pH and skin diseases, such as atopic dermatitis, acne vulgaris and xerosis (dry skin). Although the relationship is complex, research into our skin’s physiological make-up has shown that the acidic nature of the ‘stratum corneum’ (the outermost layer of our skin) can impact our skin’s integrity, antimicrobial defense mechanisms and the protective function of our skin barrier [5,6].
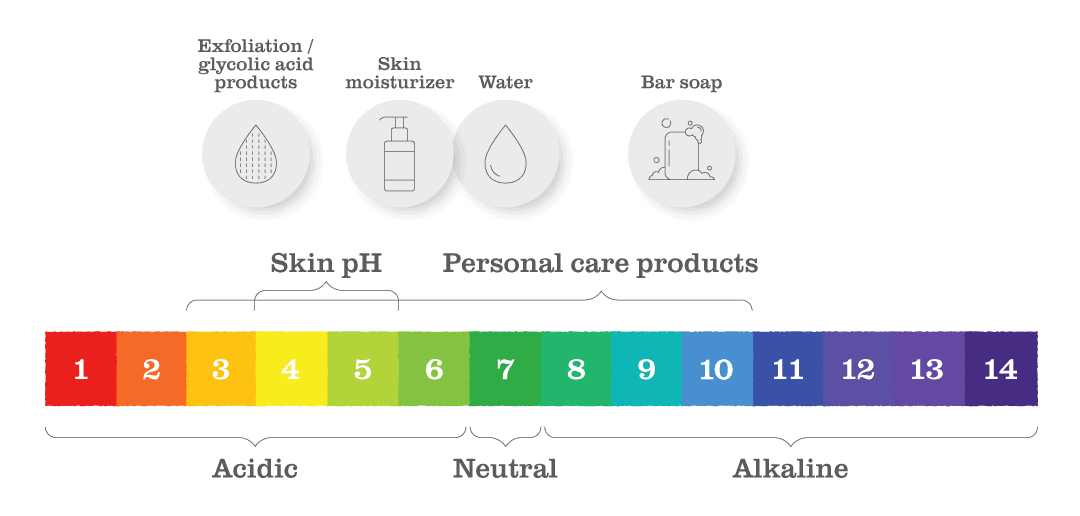
Fig. 1 | The normal range for skin surface pH is acidic at 4.1–5.8, with slight variations at different points across the body. Contemporary hygiene practices and products, such as soap and detergents, can impact the skin pH — mediated in part by the microbiome — and can play a role in skin disease and allergies.
Skin pH balance
Skin pH depends largely on several physiological factors (as well as environmental impacts), but key traits responsible for determining pH levels are age and skin buffer capacity. Maintaining a normal skin pH is key for the physical functioning of our skin (for example, when it comes to skin barrier function), and here the microbiome also plays a part.
Age. Skin pH varies with age. Newborns do not have a fully formed skin and typically have elevated pH. During the first year of life, as well as strengthening of the skin barrier, infant skin acidifies [7]. Once this acidification has occurred, the skin pH remains fairly constant until the
fifth decade of life, where studies have shown skin pH to increase in postmenopausal women (linked to changes associated with decreasing oestrogen production) and similarly in both elderly males and females [8–15].
Buffer capacity. The skin has its own remarkable ability to stabilize its pH, which is known as its buffer capacity. This refers to the ability of the skin to resist acidic or alkaline assaults that threaten to take the skin pH beyond its usual levels. Buffer capacity of the skin is reduced in both infant and elderly skin, which may explain why these individuals within these age groups show more sensitivity to detergents and other irritants [16,17]. The skin’s ability to buffer itself against pH insults is fairly substantial, but repeated washing with products such as alkaline soap can reduce this capacity by washing away its inherent buffering components [17].
Skin barrier function
The stratum corneum — the outer layer of the skin — is the main layer controlling skin barrier function. It results from a complex differentiation process (the process in which one cell type changes to another) of skin cells known as keratinocytes, which change to corneocytes (see Fig. 2). The makeup of the stratum corneum is comparable to a brick wall of cross-linked lipids and proteins that acts as a highly effective membrane to protect against the onslaught of environmental factors, such as dehydration stress and pollution. When you consider that the stratum corneum is only 15–20 cm thick, its function as a membrane is an incredible result of human evolution.
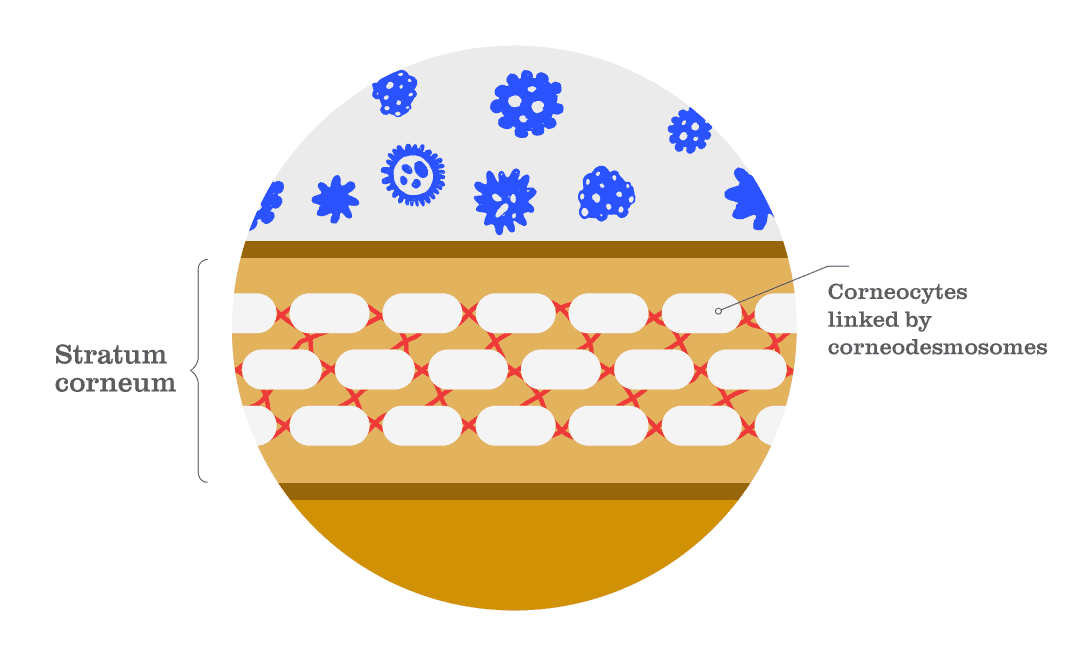
Fig. 2 | The stratum corneum is the outermost layer of the skin. It consists of ‘corneocytes’ (a type of skin cell) supported by ‘corneodesmosomes’ (a type of skin protein) and other crosslinked lipids and proteins. These components form a wall that acts as a highly effective barrier to protect against the external environment and threats such as dehydration and pollution.
Acidic pH of the skin surface is well recognized as a key regulating factor when it comes to maintaining the delicate conditions of the stratum corneum [18] . Within this, the health and function of the skin barrier is largely dependent on the complex interaction between pH and a protein known as filaggrin, as well as keratinocyte cells and a host of lipid- and protein-processing enzymes, and the microbiome — all of which are controlled by pH level.
Filaggrin. Filaggrin is found inside keratinocyte cells. The breakdown of filaggrin leads to acidification of the skin and a reduction in skin pH, highlighting its role as our skin’s buffer. When our skin is assaulted with water and detergents — which alkalize the skin, leading to a damaged skin barrier — more filaggrin is produced to lower the skin pH and repair the skin barrier [19]. The importance of filaggrin is also made clear due to the link between this protein and the worsening of our skin health — filaggrin gene mutations are, for example, a significant risk factor for the development of atopic dermatitis [20].
Lipid- and protein-processing enzymes. If we continue to build a ‘bricks and mortar’ model, the mortar supporting the stratum corneum is composed of various lipids produced from layered structures within keratinocyte cells. The enzymes responsible for producing these lipids need an acidic or low pH to function. For instance, these enzymes synthesize ceramides, which are critical to the permeability function of our skin barrier [21].
Outer skin barrier function is also highly dependent on a group of enzymes known as ‘serine proteases’, which are regulated by pH and responsible for cleaving peptide bonds in proteins. Skin proteins called ‘corneodesmosomes’ bind skin cells together — if you consider the brick wall analogy, these proteins can be thought of as “masonry tiles that act as molecular rivets between the bricks in a three-dimensional space” [22]. It is necessary to cleave these masonry tiles for our skin to shed [23]. Increases in skin pH lead to increases in serine protease activity as has been observed in dry skin, dermatitis and, most importantly, skin barrier dysfunction caused by surfactants (a primary component of cleaning detergents) and other environmental assaults [24].
Microbiome. The last factor associated with a healthy skin barrier is the skin microbiome. It is recognized that commensal skin microbes (that is, microbes that are beneficial or provide a protective function) help contribute to a healthy acidic skin pH [25–27]. For example, Cutibacterium acnes bacteria can influence the levels of some skin components; this includes reducing triglycerides in sebum (the waxy protective coating on our skin surface) but not the levels of free fatty acids, which differ in structure and function to the triglycerides and increase the acidity of the skin. Many pathogenic microorganisms, such as Staphylococcus aureus and Streptococcus pyogenes, are inhibited by the skin’s acid pH, but an acidic pH also favours the growth of Staphylococcus epidermidis and Corynebacterium bacteria, which are considered part of the normal skin flora [28,29].
Impact of cosmetics and cleansers on skin pH
Contemporary hygiene practices that are part of our social culture, like bathing daily with water, soap and detergents, can negatively impact skin pH. Subsequently, such practices and products are thought to play a part in increased rates of skin conditions such as atopic dermatitis, and have also been linked to increases in vulnerabilities to food allergies [30].
Research has demonstrated, for example, that detergents and surfactants damage the skin barrier, and that surfactants cannot determine the difference between skin debris and skin lipids [31]. Small and repeated pH increases from daily soap-based cleanser use has also been shown to reduce skin barrier repair[32], and when one group of researchers measured the pH of many commonly used cleansers, a correlation was found between the pH of cleansers and skin irritation — the higher the pH the more irritation [33].
As shown in Fig. 1, soap is typically alkaline, with a pH of approximately 10. It is also worth noting that not all cleansers are soap, offering alternatives that may be better suited. Syndet cleansers are the most used cleansers today and are composed of synthetic detergents known as syndets. These cleansers have a pH formulated between 5 and 7, which is closer to normal skin pH.
Although the skin microbiome has been shown to be fairly resilient in healthy individuals, the effects of cosmetics and cleansers, as well as antiseptic or antibiotic use, may also influence skin microbiome diversity. The significance of such effects is not yet clear but, for example, cosmetic ingredients such as lactic acid, citric acid and sorbic acid that help maintain an acidic environment may also help support a healthy skin microbiome [18].
These relationships indicate that topically applied products could contribute to maintenance of a healthy skin condition via skin pH control. Formulators, dermatologists and us as consumers would benefit from more precise guidance on favourable product pH values and the selection of cosmetic ingredients that could be responsible for lowering pH, together with a better understanding of the mechanisms that underlie the process of skin acidification (including the role of the microbiome).
Concluding remarks
There is, therefore, a fine balancing act to achieving good hygiene while protecting the integrity of the skin’s natural barrier. The chemical reaction between detergent, water and skin, for instance, is complex; and although the skin microbiome has been shown to have a fair amount of the resilience, the full impact of this balancing act on the skin pH and microbiome is not yet fully understood.
However, it is clear that skin pH is integral to the skin’s ability to function as a permeable barrier. Contemporary hygiene practices, such as bathing daily with water, soap and detergents, may negatively impact the skin pH — mediated in part by the microbiome — and can play a role in skin disease and allergies.
Hear from more of our experts in the Views from section on the Content Hub and follow us on Instagram to receive the latest updates.
References
1. Segger, D. et al. Multicenter study on measurement of the natural pH of the skin surface. Int. J. Cosmet. Sci. 30, 75 (2008).
2 Lambers, H. et al. Natural skin surface pH is on average below 5, which is beneficial for its resident flora. Int. J. Cosmet. Sci. 28, 359–370 (2006).
3. Kleesz, P. et al. Full-body skin mapping for six biophysical parameters: baseline values at 16 anatomical sites in 125 human subjects. Skin Pharmacol. Physiol. 25, 25–33 (2012).
4. Prescott, S. L. et al. The skin microbiome: impact of modern environments on skin ecology, barrier integrity, and systemic immune programming. World Allergy Organ J. 10, 29 (2017).
5. Schade, H. & Marchionini, A. Der Säuremantel der Haut nach Gaskettenmessngen. Klin. Wochenschr. 7, 12–14 (1928).
6. Hachem, J. P. et al. pH directly regulates epidermal permeability barrier homeostasis, and stratum corneum integrity/cohesion. J. Invest. Dermatol. 121, 345–353 (2003).
7. Nikolovski, J. et al. Barrier function and water-holding and transport properties of infant stratum corneum are different from adult and continue to develop through the first year of life. J. Invest. Dermatol. 128, 1728–1736 (2008).
8. Joseph, N. et al. Titration curves of human epidermis in relation to age. Federation Amer. Soc. Exp. Biol. 16, 9650 (1957).
9. Choi, E.-H. et al. Stratum corneum acidification is impaired in moderately aged human and murine skin. J. Invest. Dermatol. 127, 2847–2856 (2007).
10. Man, M. et al. Variation of skin surface pH, sebum content and stratum corneum hydration with age and gender in a large Chinese population. Skin Pharmacol. Physiol. 22, 190–199 (2009)..
11. Schreml, S. et al. Impact of age and body site on adult female skin surface pH. Dermatology 224, 66–71 (2012).
12 Luebberding, S. et al. Age-related changes in male skin: quantitative evaluation of one hundred and fifty male subjects. Skin Pharmacol. Physiol. 27, 9–17 (2014).
13. Levin, J. & Maibach, H. Human skin buffering capacity: an overview. Skin Res. Technol. 14, 121–126 (2008).
14. Levin, J. & Maibach, H. pH buffering considerations in mature skin. Cosmet. Toilet. 126, 422–428 (2011).
15. Luebberding, S. et al. Age-related changes in skin barrier function–quantitative evaluation of 150 female subjects. Int. J. Cosmet. Sci. 35, 183–190 (2013).
16. Thune, P. et al. The water barrier function of the skin in relation to the water content of stratum corneum, pH and skin lipids. The effect of alkaline soap and syndet on dry skin in elderly, nonatopic patients. Acta Derm. Venereol. 68, 277–283 (1988).
17. Ayer. J. & Maibach, H. I. Human skin buffering capacity against a reference base sodium hydroxide: in vitro model. Cutan. Ocul. Toxicol. 27, 271–281 (2008).
18. Lukic, M. et al. Towards optimal pH of the skin and topical formulations: from the current state of the art to tailored products. Cosmetics 8, 69 (2021).
19. Rawlings, A. V. et al. Stratum corneum moisturization at the molecular level. J. Invest. Dermatol. 103, 731–741 (1994).
20. Margolis, D. V. et al. The persistence of atopic dermatitis and filaggrin [FLG] mutations in a US longitudinal cohort. J Allergy Clin Immunol 130, 912–917 (2012).
21. Rippke, F. et al. The acidic milieuof the horny layer: New findings on the physiology and pathophysiology of skin pH. Am. J. Clin. Dermatol. 3, 261–272 (2002).
22. Rawlings, A. V. Recent advances in skin ‘barrier’ research. J. Pharm. Pharmacol. 62, 671–677 (2010).
23. Borgono, C. A. et al. A potential role for multiple tissue kallikrein serine proteases in epidermal desquamation. J. Biol. Chem. 282, 3640–3652 (2007)
24. Rawlings, A. V. Stratum Corneum proteases and dry skin conditions. Cell Tissue Res. 351, 217–235 (2013).
25. Grice. E. & Segre, J. The skin microbiome. Nat. Rev. Microbiol. 9, 244–253 (2011).
26. Roth, R. R. & James, W. D. Microbial ecology of the skin. Annu. Rev. Microbiol. 42, 441–464 (1988).
27. Elias, P. M. The skin barrier as an innate immune element. Semin. Immunopathol. 29, 3–14 (2007).
28. Gribbon, E. M. et al. Interaction ofPropionibacterium acneswith skin lipidsin vitro. J. Gen. Microbiol.
29. Hentges, D. J. The anaerobic microflora of the human body. Clin. Infect. Dis. 16, S175–S180 (1993).
30. Cork, M. et al. Epidermal barrier dysfunction in atopic dermatitis. J. Invest. Dermatol. 129, 1892–1908 (2009).
31. Dykes, P. Surfactants and the skin. Int. J. Cosmet. Sci. 20, 53–61 (1998).
32. Fluhr, J. W. et al. Generation of free fatty acids from phospholipids regulates
stratum corneum acidification and integrity. J. Invest. Dermatol. 117, 52–58 (2001).
33. Baranda, L. et al. Correlation between pH and irritant effect of cleansers marketed for dry skin. Int. J. Dermatol. 41, 494–499 (2002).